We are an electroanalytical chemistry research lab, that is highly interdisciplinary and collaborative. Our active research projects cover the fields of nanoscience, biosensing, chemical imaging and materials.
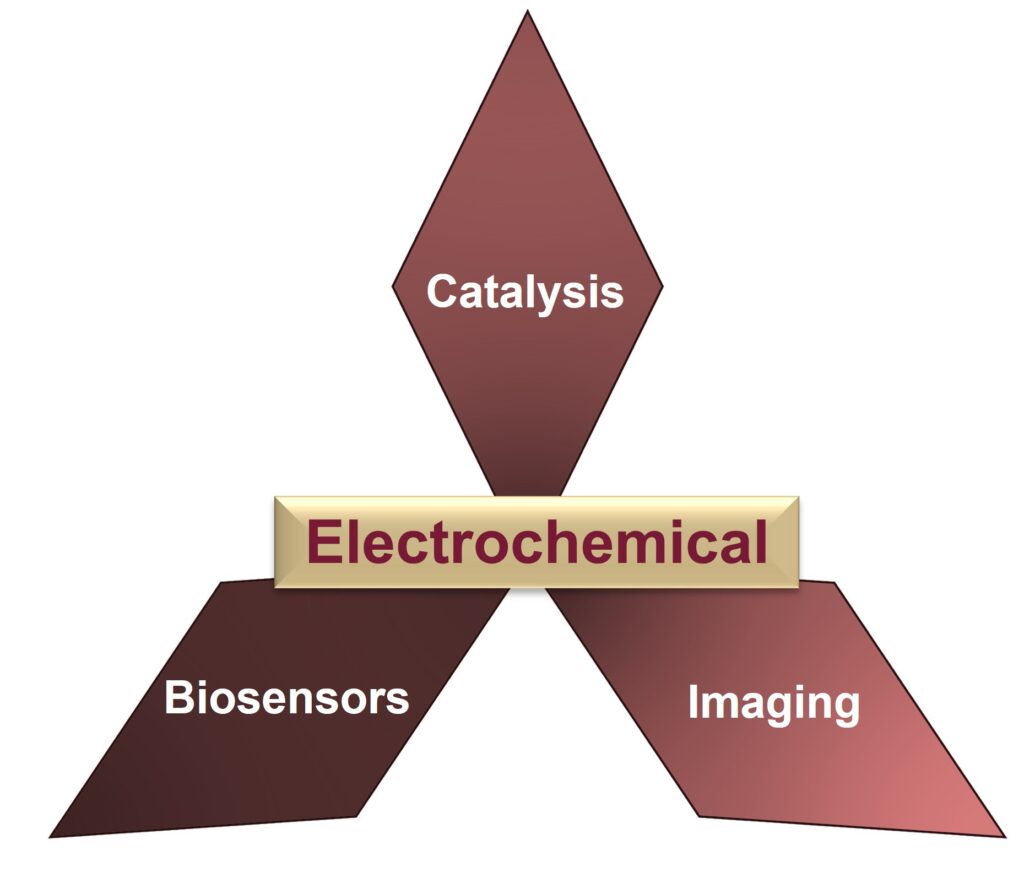
Multianalyte detection using aptasensors and microelectrode arrays
Electrochemical aptamer-based (E-AB) sensors typically use artificial single-stranded DNA or RNA aptamers that are immobilized on an electrode surface, with a redox active tag such as methylene blue (MB) appended to the molecule. Aptamers have the ability to specifically bind to target analytes such as metabolites, toxins and biomarkers, with high affinity. We use aptamers that undergo a conformational change when binding to their analyte, that induces a change in current signal that allows quantitative detection. This change in redox current arises due to the change in electron transfer rate between MB and the supporting electrode, to which the aptamer is tethered. This biosensing mechanism offers high sensitivity and selectivity in detecting a wide range of analytes, from biomarkers in medical diagnostics to environmental pollutants and foodborne pathogens. As such, this class of sensor has found extensive application in healthcare, environmental monitoring, and food safety, providing rapid and accurate detection methods for improving public health and safety.
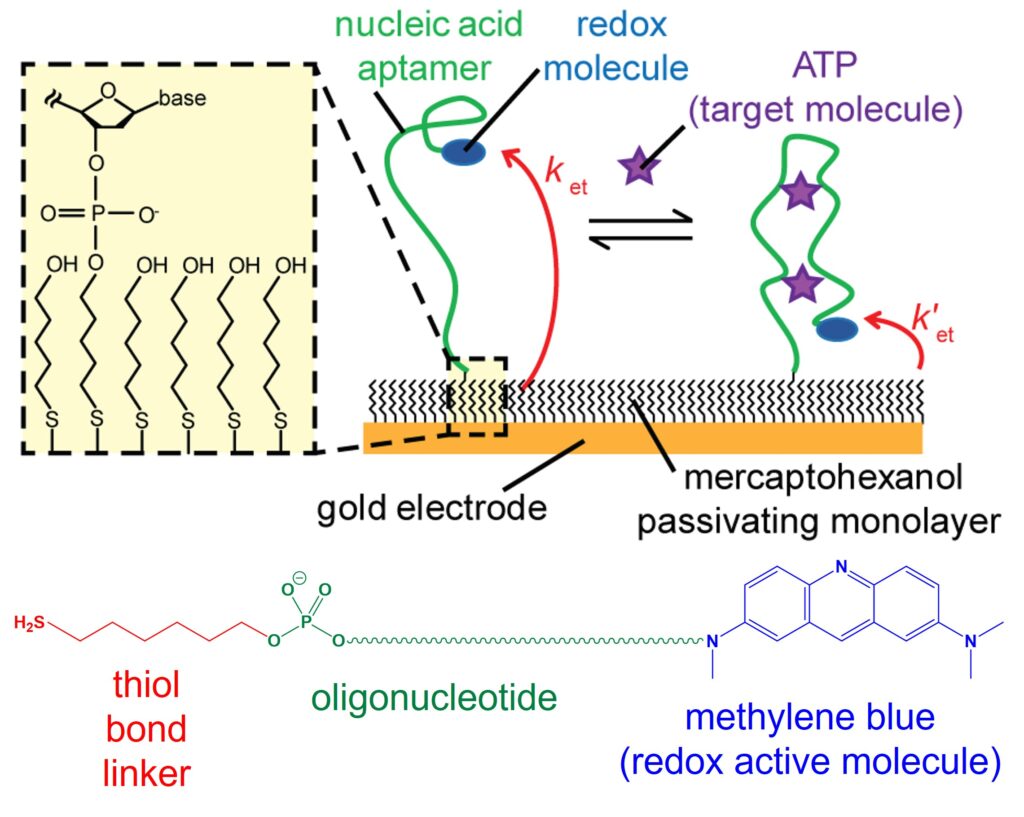
We are designing and fabricating microelectrode arrays, of two to seven closely spaced electrodes, to simultaneously detect multiple analytes using the E-AB sensor platform. We have recently demonstrated the selective aptamer modification of Au surfaces in a microelectrode sensor array, that enables multiplexed detection. We are currently working on using this biosensor array platform to measure localized analyte concentrations, in conjunction with scanning electrochemical microscopy (SECM). Furthermore, our research agenda extends to enhancing the performance of the E-AB sensor platform on a microscale electrode, which involves control of the morphology of electrodeposited gold nanostructures. These concerted efforts collectively aim to advance E-AB sensing technology, for microscale chemical mapping of multiple analytes.
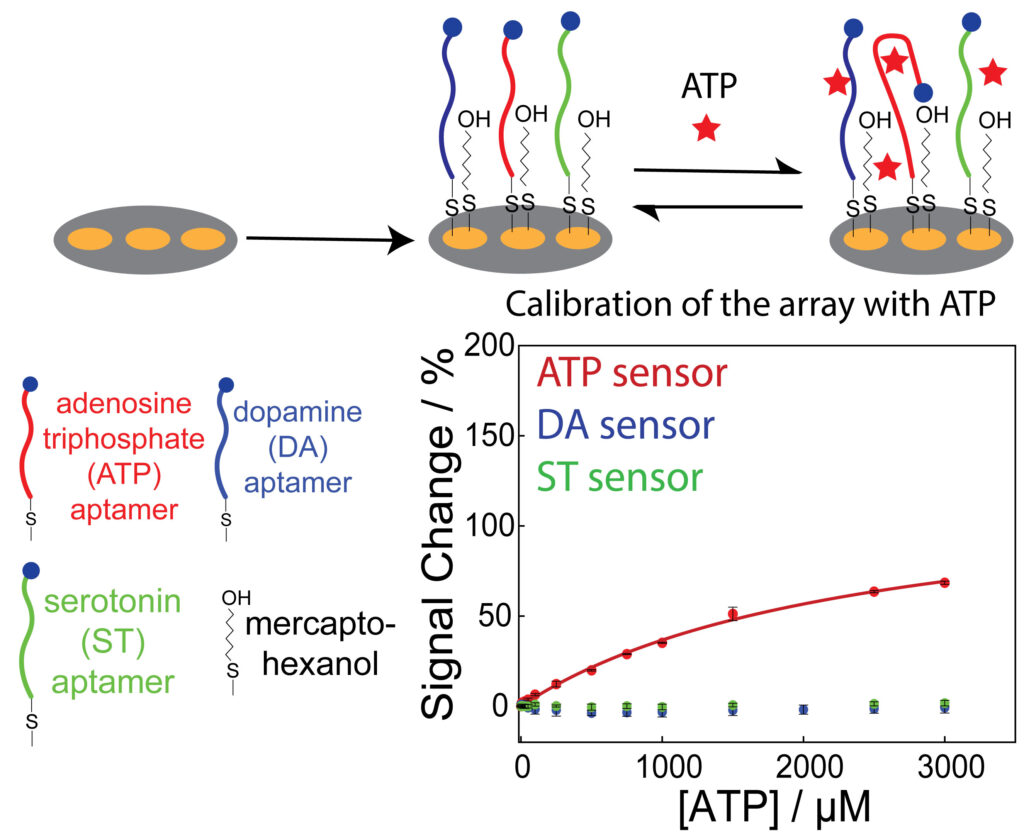
Relevant publications:
Selective aptamer modification of Au surfaces in a microelectrode sensor array for simultaneous detection of multiple analytes. D. Sen and R. A. Lazenby, Anal. Chem. 2023, 95, 17, 6828–6835.
Electrochemical biosensor arrays for multiple analyte detection. D. Sen and R. A. Lazenby, Anal. Sens. 2023, e202300047.
Controlling gold morphology using electrodeposition for the preparation of electrochemical aptamer-based sensors. A. J. Ritz, O. Stuehr, D. Comer, and R. A. Lazenby, ACS Appl. Bio Mater., 2024, 7, 1925–1935.
Scanning ion conductance microscopy of living cells
Scanning ion conductance microcopy (SICM) is a scanning probe technique used for cell topography imaging. The technique uses ion current as a feedback mechanism for maintaining a constant tip-sample separation distance during imaging. An ion current is generated by applying a positive voltage to a Ag/AgCl quasi-reference counter electrode (QRCE) inside the nanopipette, vs a second QRCE outside the nanopipette. This bias results in the movement of anions (e.g. Cl- ions) inside the nanopipette, and cations (e.g. K+ and Na+ ions) outside the nanopipette. When the nanopipette is translated towards the substrate, such as a cell membrane, the ion current decreases. This experiment is known as an approach curve. To image a cell, a decrease in ion current that is as small as 1% less than the maximum current away from the substrate is used as a feedback setpoint, to sense the surface and prevent the nanopipette from touching the cell. The nanopipette is then moved in a raster manner in a hopping mode to scan the cell topography. SICM has offered a platform for label free and non-invasive live imaging of cell topography, in contrast to the standard method of cell topography measurement such as scanning electron microscopy (SEM), which is not compatible for live cell imaging.
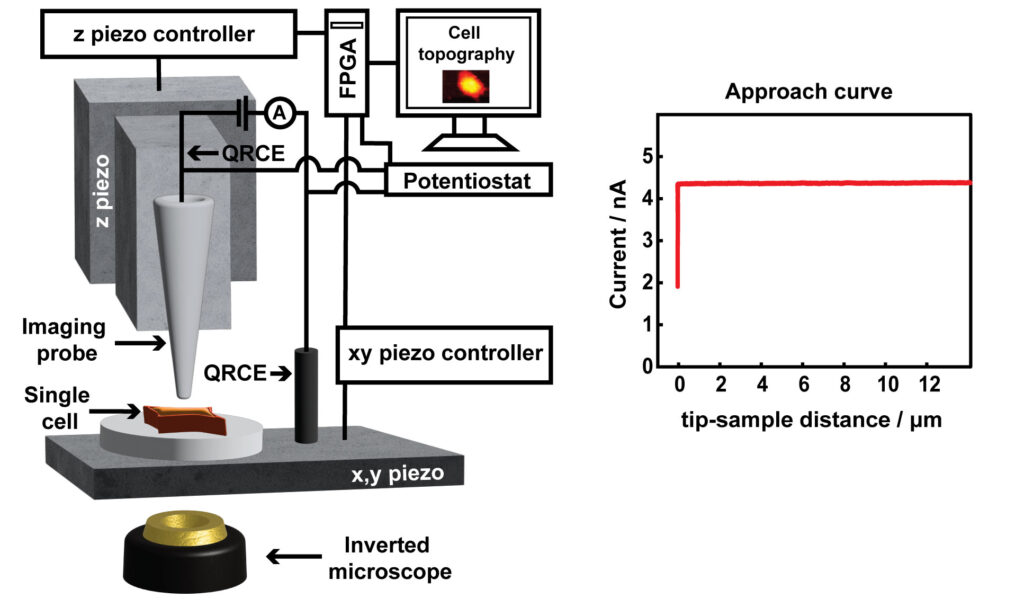
SICM has been used for a wide variety of studies such as imaging the interaction of viral like particles with the cell membrane, studying endocytosis, and dynamic changes in the morphology of platelets. In our lab, we are using SICM for the measurement of the topography of single A549 cells. Specifically, the studies of apoptosis induced by anticancer drugs, the development of nanoscale pH sensors for the pH imaging at single cells, and the study of microparticle interactions with cells.
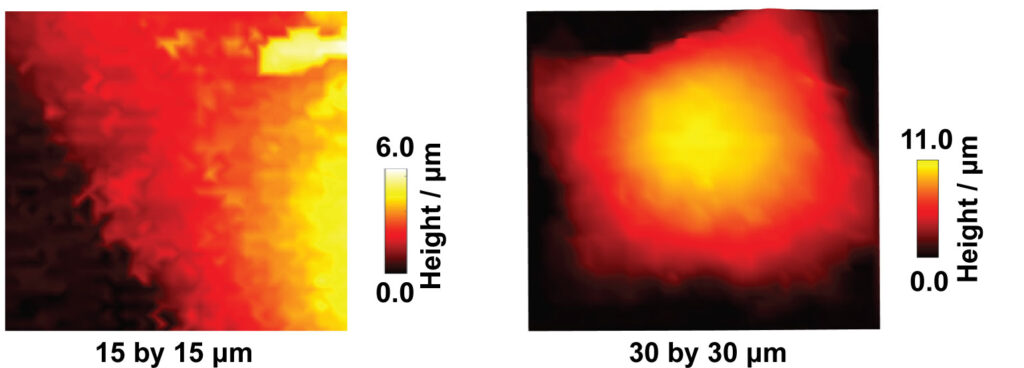
Relevant publications:
Scanning ion conductance microscopy revealed cisplatin-induced morphological changes related to apoptosis in single adenocarcinoma cells. Y. Muhammed and R. A. Lazenby, Anal. Methods, 2024, 16, 503–514.
Nanopores and nanopipettes for chemical sensing
Nanopores are revolutionary tools in the field of chemical sensing, allowing for the detection and analysis of molecules at the nanoscale. Among the various nanopore-based platforms, nanopipettes and nanoporous membranes are known for their ability to facilitate precise and selective chemical sensing. These minuscule apertures, often measuring just a few nanometers in diameter, act as gateways for molecules to travel through, providing a unique platform for studying various analytes, ranging from ions and small molecules to macromolecules like proteins and DNA. The utilization of nanopores in chemical sensing enables rapid and sensitive detection while also holding immense promise in areas such as single-molecule analysis, drug screening, and biomarker identification. Nanopipettes and nanoporous membranes hold the potential to deepen our understanding of molecular dynamics, enabling us to unravel intricate chemical processes and develop cutting-edge sensing applications with extraordinary sensitivity and selectivity.
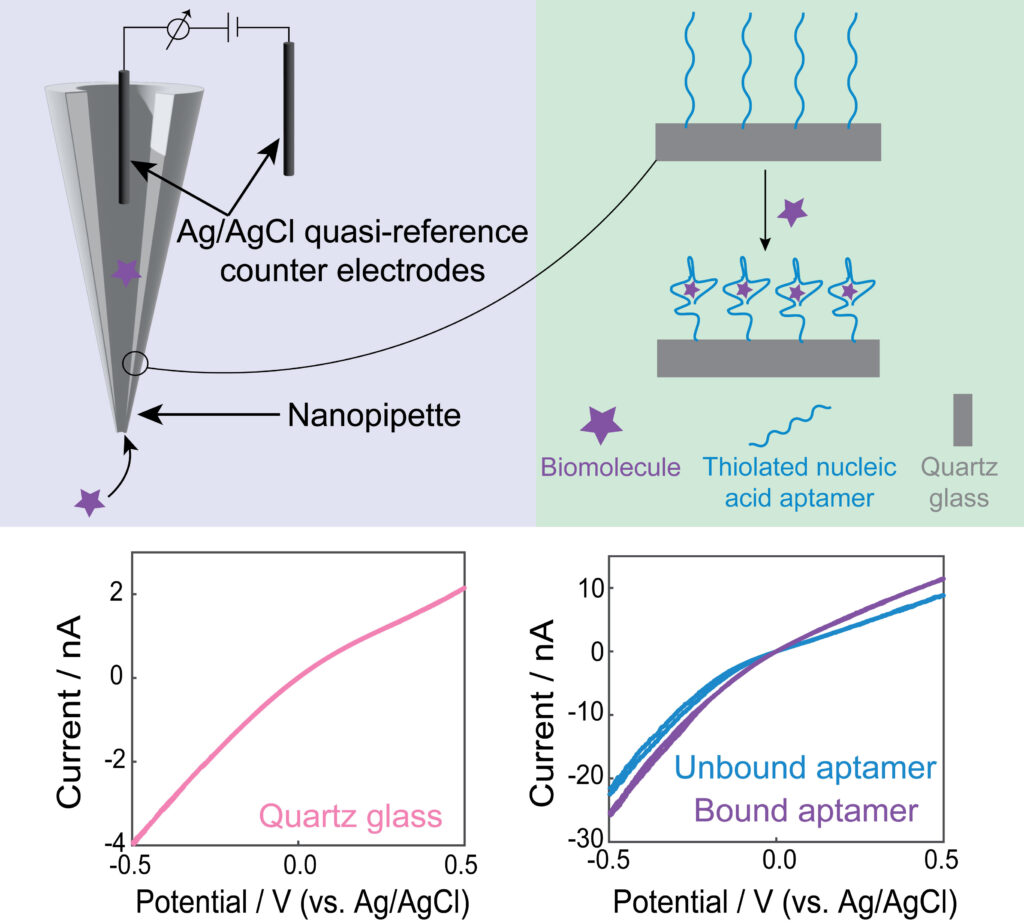
Our lab functionalizes nanopores with aptamers, which are short single-stranded DNA or RNA sequences selected for their high specificity to target molecules. This functionalization creates a tailored molecular recognition interface within the pore that allows for selective capture and detection of specific analytes. The study of ion current rectification within these aptamer-modified nanopores has proven instrumental. When a target molecule binds to the aptamer inside the nanopore, it can alter the ion flow through the pore, leading to distinctive changes in the recorded electrical signals. By analyzing the recorded electrical signals, we can gain a deeper understanding of binding effects and optimize biosensing in the nanoscale.
Scanning electrochemical microscopy
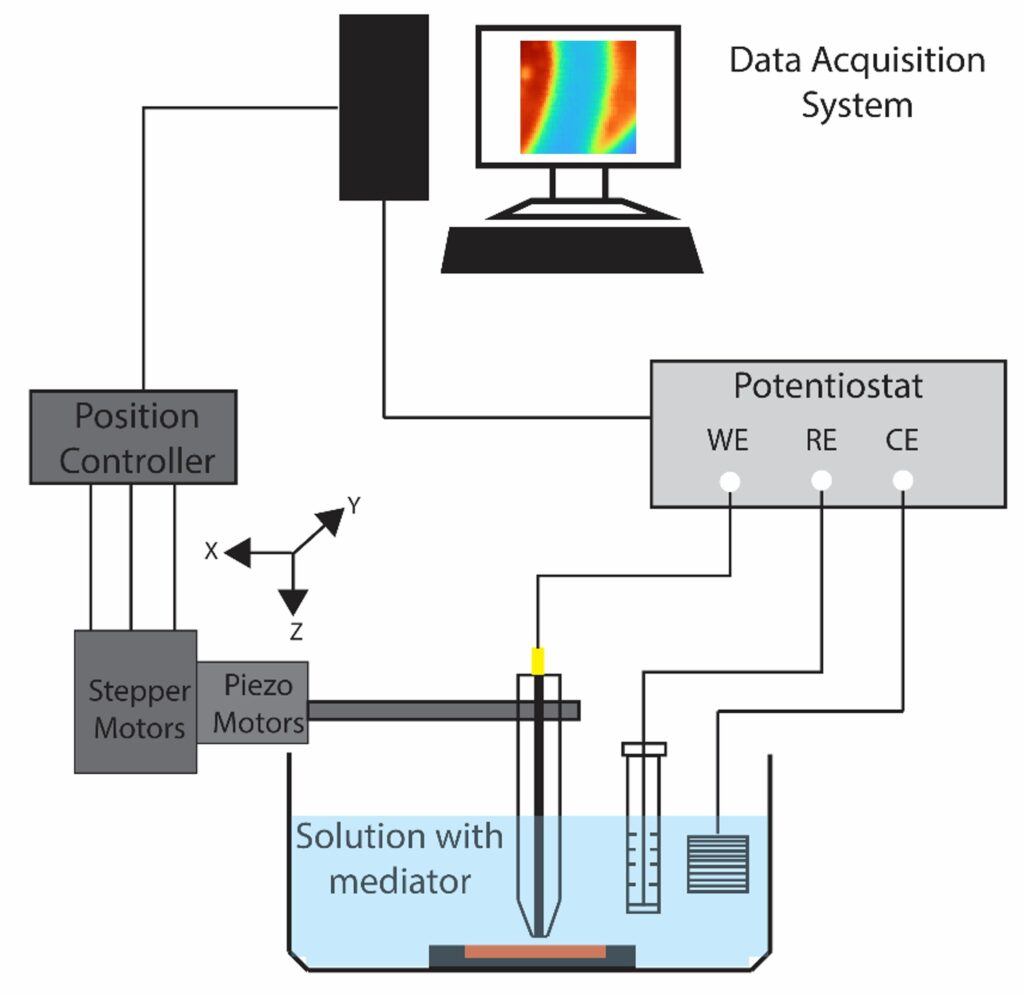
Scanning electrochemical microscopy (SECM) is designed to probe the local electrochemical behavior of a sample surface by employing a small-scale electrode, typically in the range of micro to nano meter sizescale. The electrode is scanned in close proximity to the sample, while simultaneously recording electrochemical response. This process allows us to generate high-resolution maps of various electrochemical phenomena, such as local reactivity, redox species concentration gradients, and ion transport profiles. The versatility of SECM extends to a wide range of applications, making it invaluable for understanding corrosion processes, exploring the intricacies of biological systems, characterizing electrode materials, and mapping ion transport in semiconductors. Additionally, SECM provides insights into reaction kinetics and the local electroactivity of materials, making it a useful tool across multiple scientific disciplines.
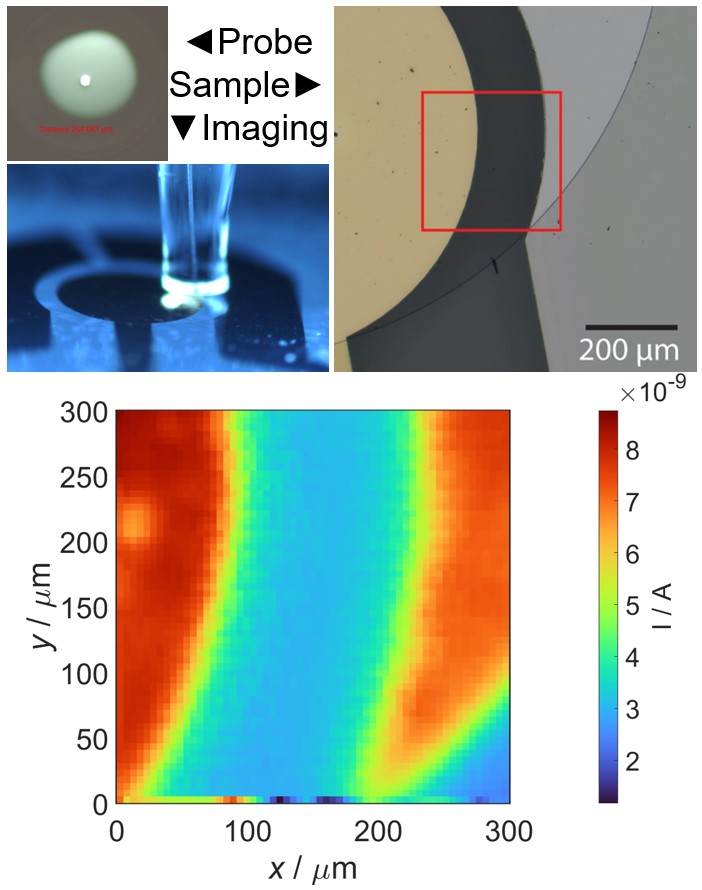
Non-oxide electrocatalysts for the oxygen evolution reaction
The design of efficient electrocatalysts for the water-splitting reaction are pivotal in driving sustainable energy technologies like water electrolyzers, fuel cells, and metal-air batteries, particularly in the context of the oxygen evolution reaction (OER). OER is a sluggish four-electron half-reaction that is responsible for produces oxygen gas from water at the anode and restricts the rate of overall water-splitting. To address its unfavorable kinetic limitations, researchers are actively developing electrocatalysts that can reduce the energy barrier, thus improving the efficiency of oxygen gas production while minimizing overpotentials.
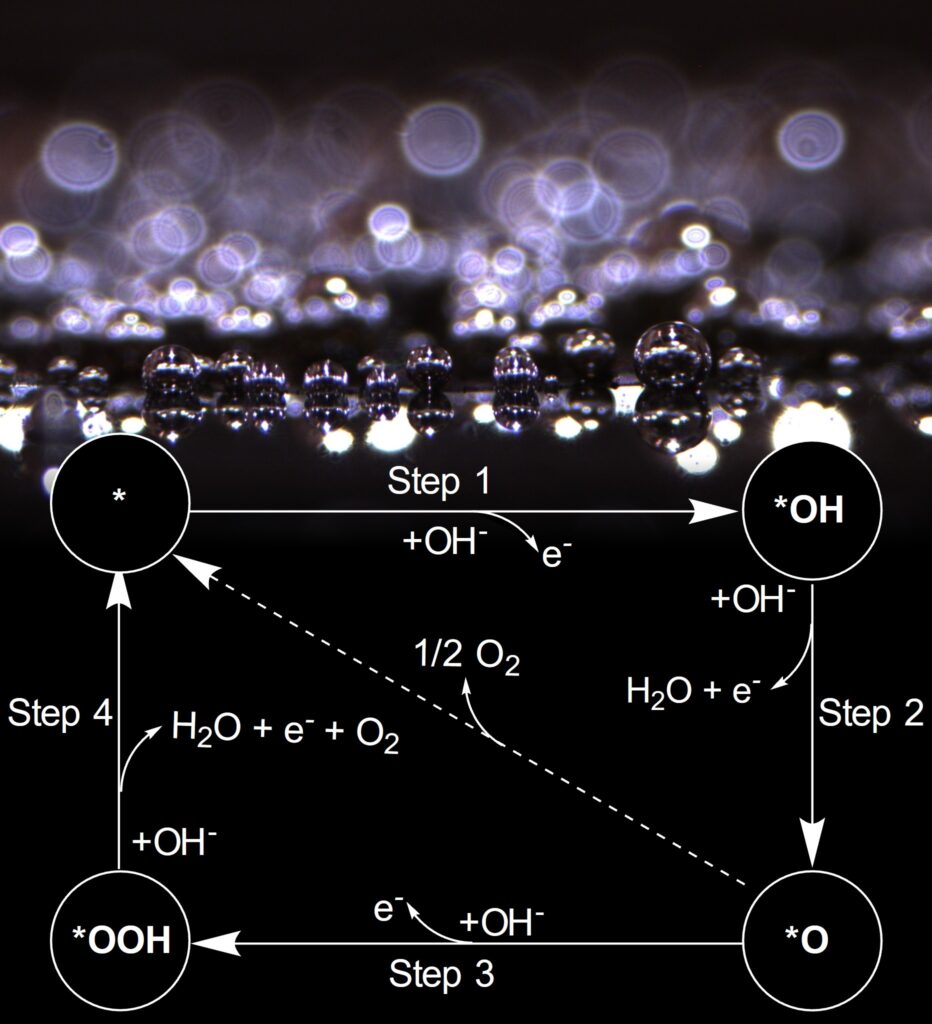
Traditionally, ruthenium and iridium oxides have been considered the benchmarking standards for OER catalysis, despite their drawbacks, such as high cost and limited availability due to their low abundance. In the search for more cost-effective and sustainable alternatives, various materials, including oxyhydroxides, transition metal oxides, perovskite oxides, spinel oxides, and alloys, have gained attention as potential OER catalysts. Additionally, transition metal non-oxide-based electrocatalysts, like sulfides, phosphides, and carbides, have emerged as promising contenders. These materials offer better electrical conductivity and attractive electrocatalytic performance, making them viable alternatives to oxide-based catalysts. Our laboratory, in particular, is focused on understanding the electrochemical performance of transition metal-based carbides due to their potential for scalable, cost-effective production and impressive performance in the hydrogen evolution reaction (HER), making them intriguing candidates for bifunctional HER and OER applications.
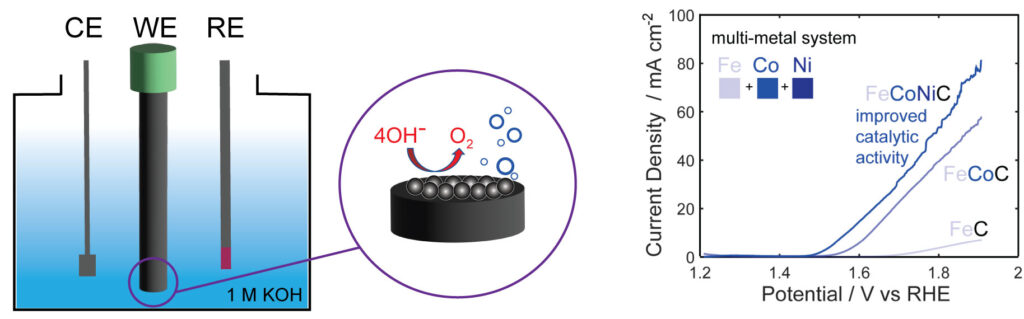
Harnessing the synergistic effects of various transition metals, multimetallic catalysts offer a solution to the limitations associated with single-metal catalysts. Specifically, these catalysts have proven their ability to fine-tune electrocatalytic activity through adjustments in electronic structure, lattice and surface strain, and the exposure of more abundant active sites for catalysis. However, in multimetallic non-oxide-based catalysts even more dynamic changes are observed due to surface oxide reconstruction under challenging electrochemical conditions. This phenomenon can significantly influence optimal surface-active sites and modulate electrocatalytic activity, and these effects are not well understood. Therefore, understanding and controlling the reconstruction process are imperative for tailoring nanocarbide catalysts to achieve efficient OER performance in water electrolysis conditions. Our research endeavors to pinpoint and comprehend how oxide growth can either enhance or suppress the OER in carbide nanomaterials based on material composition and transition metal ratios.
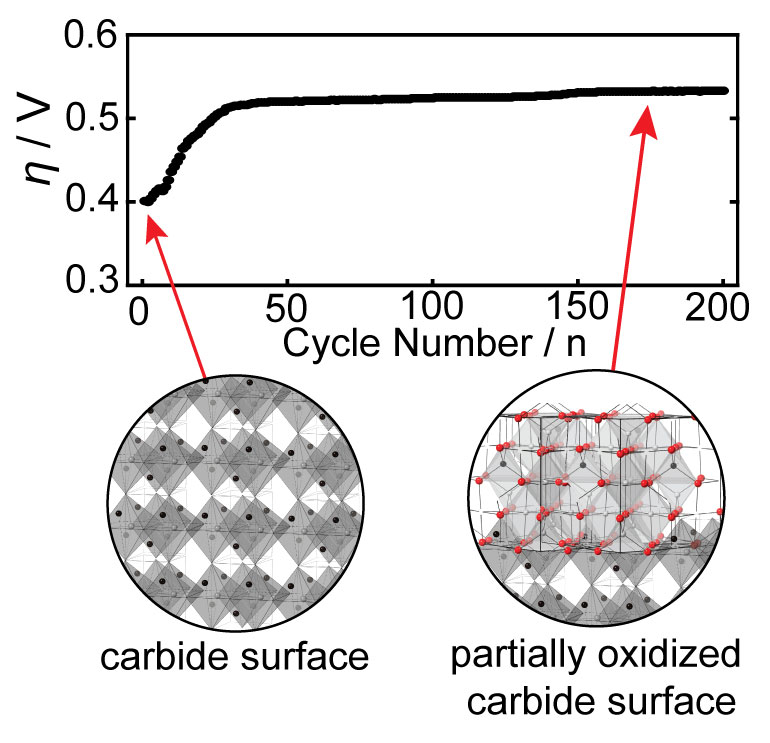
Relevant Publications:
Electrocatalytic activity and surface oxide reconstruction of bimetallic iron-cobalt nanocarbide electrocatalysts for the oxygen evolution reaction. A. J. Ritz, I. A. Bertini, E. T. Nguyen, G. F. Strouse and R. A. Lazenby, RSC Advan. 2023, 13, 33413–33423.
A single source, scalable route for direct isolation of earth abundant nano-metal carbide water splitting electrocatalysts. E. T. Nguyen, I. A. Bertini, A. J. Ritz, R. A. Lazenby, K. Mao, J. R. McBride, A. V. Mattia, J. E. Kuszynski, S. F. Wenzel, S. D. Bennett and G. F. Strouse, Inorg. Chem., 2022, 61, 13836–13845.